Juan David's Newsletter - October 23rd, 2022
Flight Forces (with an in-depth exploration of the Mystery of Lift)
Morning,
I spent the entire week at the Wolfram tech conference where I met lots of interesting people and created a cool project using the James Webb Telescope API (will share once I’m done).
I’m sharing an essay about the forces of flight with a detailed explanation of lift. This will be the 9th essay I’ve shared so far in this flying series.
Flight Forces (with an in-depth exploration of the Mystery of Lift)
This essay draft is part of an upcoming mega essay (or book) of an exploration of teaching myself EVERYTHING on Flying from Hidden History to Hardcore Engineering.
In this series, we’ve talked deeply about the history of flying because that is my tendency when I’m learning about new topics. I’ve realized when I learn the history of a topic, I can fully understand why things are the way they are. But after learning the history, my next instinct is learning the mathematics and engineering behind it all.
This essay descends deeply into the forces of flight.
Learning history is important because it gives us context. Once we have context, we can understand better and learn faster.
George Cayley was the first person to understand the forces of flight.
What are these four things, and why are they important? They are the four forces of flight. To figure out how to make something fly, you must consider them.
These forces push a plane up, down, forward, or slow down. These forces apply to all flying things, from frisbees to planes. Well, things fly because of the four forces.
Let’s define them:
Thrust is a force that moves an aircraft in the direction of the motion.
Drag is the force that acts opposite to the direction of motion.
Weight is the force caused by gravity.
Lift is the force that holds an airplane in the air.
These forces are all related and determine how flying things behave in the air.
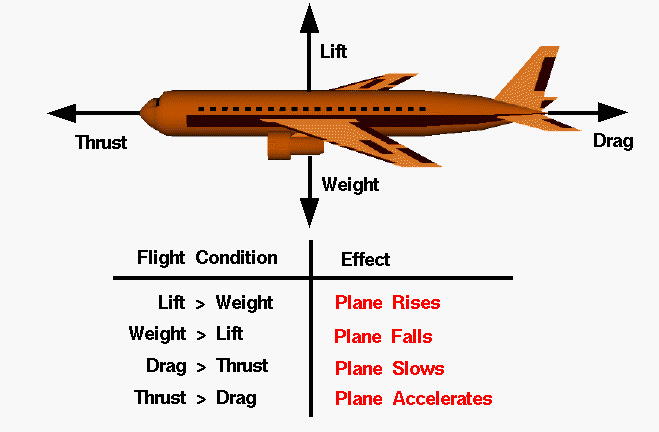
The art of flying can be defined as the most beautiful, careful, give-and-take relationship. For the whole thing to work, the different forces must equilibrate each other to take off, land, accelerate, or slow down.
The lift balances the weight while the thrust exceeds the drag for the plane to keep itself in the air by using the engine for thrust and wings for lift.
For an airplane to fly, there are two types of forces. Forces that act on the plane (weight and drag) and forces generated by the plane (thrust and lift). Flight forces are like dancing tango, and you need to be careful not to step on each other.
You'd get heavier-than-air aircraft when you figure out the proper equilibrium for these forces.
Heavier-than-air aircraft? At first, I had no idea what this meant, but it's worth talking about because it brings us into an essential rabbit hole.
Heavier-Than-Air vs Lighter-Than-Air
We have heavier-than-air and lighter-than-air. For instance, airplanes are heavier than air, and they fly because of the force generated by airflow over the lifting surfaces.
While balloons and airships are lighter-than-air (LTA), and they fly because they are floating in the air (buoyancy), meaning the aircraft's total weight is less than the weight it displaces.
The Wright Brothers accomplished what everyone thought was impossible: the first-ever heavier-than-air aircraft with the Wright Flyer.
First, let's start with heavier-than-air flying things. How on Earth do they work? Brilliant people didn't think it would work. William Thomson, the guy who formulated the first and second laws of thermodynamics, thought it was impossible.
But for some reason, the Wright Brothers figured it out. Just think about how hard this is. You need to overcome motherf*******'s gravity to make things fly, and gravity is just evil, man.
So how do you overcome gravity? Understanding the flying forces.
Flying Forces: Lift, Drag, and Pitch
Lift
For anything to rise into the air, you need a force that equals or exceeds the force of gravity. This force is called lift. In heavier-than-air machines, the lift is created by airflow over an airplane wing.
The airfoil's shape causes air to flow faster on top than on the bottom, and this fast-flowing air decreases the surrounding air pressure. When the air pressure is greater below the airfoil than above, a resulting lift force is created!!!!!
The physicist Juan Malcadena once said, “It is often said that a picture is worth a thousand words. A formula is worth a million pictures!"1
So we need to use formulas!!! That is the best way to describe and understand reality is with math because, as Galileo Galilei said, "Mathematics is the language in which God has written the universe."
Lift is created by the difference in pressure in the wings. When the air pressure is greater below the wing than above, we get a lift force, and by that point, you better know how to fly because you're up in the air, baby.
To represent and understand this phenomenon, we have Bernoulli's equation which is written as:
And to fully understand this equation, we need to discuss one more concept: the continuity equation. It describes the transport of some quantity:
Now that we know the Bernoulli and continuity equations, we can better understand and appreciate what causes lift. When air passes through an airfoil such as an aircraft wing, the air must "divide" in two, one going on top and the other on the bottom.
Remember, the airfoil's purpose is to reduce drag and create lift. Typically, the airfoil's shape is asymmetrical. The surface area is greater on the top than on the bottom. As air flows over the airfoil, more air displaces by the top surface than the bottom. According to the continuity law, this displacement must lead to an increase in velocity. The best way to understand this is through an example.
Imagine you are watering a garden, and you have a hose. We've all done this. You want water to flow faster, so you put your finger on the hose's outlet and get water to flow faster. Why? The path is narrower, and the velocity of the water increases.
The same effect happens with an airfoil. The large area of the airfoil's top surface narrows the path more than the bottom surface.
What about pressure? Does the pressure go up or down? According to the Bernoulli equation, when velocity increases, pressure decreases. This means that the air flowing over an airfoil will decrease in pressure. However, the pressure will increase on the bottom of the airfoil, which results in a net pressure force in the upward direction. This pressure force is called lift.
Lift: A Deeper Look
Let’s go more mathy. This isn’t to show off or to make you get confused but using more math and going deeper will radically enhance our understanding.
Lift is a force that acts in an upward direction to fight and overcome gravity. You can think of a lift as gravity’s antithesis.
But where does this force come from? What do you mean it’s a force?
A fluid (liquid, gas, or anything that flows) going through the surface of a solid object applies a force on it. This force has many parts or components, and lift is one of them. Specifically, lift is the component of the force that is perpendicular (90° degrees) to the flow direction.
As you can see, lift is just a component of this force and has a couple of other friends. But lift's best friend (or worst enemy depending on how you look at it) is the drag force because they are always together. Don't tell the drag force, but lift secretly hates drag.
Drag is the component of the surface force parallel to the flow direction. We’ll get to drag in a little bit.
To understand lift, we have less rigorous physical explanations without math and more rigorous explanations with math.
Let's start with the simplified explanations based on Newton's laws of motion or Bernoulli's principles.
Newton, are you there?
This is simple. With an airfoil, you get lift by exerting a downward force on the air. According to Newton’s third law, the air must exert an equal and opposite (upward) force on the airfoil.
Imagine you are the air, and you are flowing and flowing. As you reach the airfoil, you tend to go upwards, but when you reach the airfoil, part of you continues going up while the other component follows a downward path.
Newton would say, “Hmmmm, buddy. You need a force there.” According to Newton’s second law, this change in the flow direction required a downward force applied to the air by the airfoil. Then Newton’s third law requires the air to exert an equal and opposite upward force on the airfoil. BOOM! We get lift. With an airplane wing, the wing exerts a downward force on the air, the air exerts an upward force on the wing.
This is correct and … cute, but it's incomplete and doesn't explain the lower pressure on the top wing and other airfoil effects.
Bernoulli, are you there?
The latter explanation is based on flow detection, and the next one will be an explanation based on an increase in flow speed.
There are two common versions of the explanation: 1) “equal transit time” and 2) “obstruction” of the airflow.
The "equal transit time" explanation says air moves faster on the top surface and slower on the bottom. The reason? The path length over the upper surface is longer and must be traversed in equal transit time. Bernoulli comes in with a principle and leaves us grounded.
Bernoulli's principle says that increased flow speed is associated with reduced pressure under certain conditions, meaning the faster you go, the less pressure you have. Therefore, the reduced pressure over the upper surface results in an upward lift.
But wait a minute. What causes the flow to speed up like that?
This is a severe flaw in the equal transit time theory. This explanation is incorrect because no difference in path length is needed. Why? The assumption of equal transit is wrong because there isn't a physical principle that requires equal transit, and experimental results have shown the assumption is false.
If the “equal transit time” is wrong. What else is there? Obstruction of the airflow.
Similar to the equal transit time explanation, the "obstruction" explanation starts by arguing that flow over the upper surface is faster than the lower surface, but now the reasoning is different. It argues that the difference in speed is because the curved upper surface acts as an obstacle to the flow, forcing the streamlines to pinch closer together, making the stream tubes narrower. When this occurs, conservation of mass requires that flow speed must increase. And you know the story, we apply Bernoulli's principle, and we get lift.
However, the obstruction of flow isn't complete either. It doesn't fully explain stream tube pinching, from how it happens to why it's greater on the top surface. This makes sense for wings that are flat on the bottom and curved on top. But what about symmetric airfoils, sailboat sails, or flat plates? There's one more issue, conservation of mass doesn't explain the speed. If something speeds up, that requires an accelerating force. What is this force? This explanation does not talk about that.
So if both theories are wrong, is Bernoulli's principle wrong? No! Bernoulli's principle is correct. The issue is when people try to explain lift by arguing that a speed difference leads to a pressure difference and that the speed difference can arise from causes other than a pressure difference. It's a misunderstanding of the relationship between pressure and flow speed. Instead of one-way causation, it turns out to be a mutual interaction.
If both theories are incomplete, what explains lift?
Well, it's more complicated than we think. When the Wright Brothers flew their plane, scientists were shocked and wanted to understand the science, and that's where they applied Newton's Third Law and Bernoulli's principle. Even Einstein tried to solve this problem when he attempted to explain wing carrying capacity and proposed an airfoil design (which wasn't that good).
But there's hope. New scientific approaches using computational fluid dynamics (CFD) simulations and the Navier-Stokes equations, which fully account for air viscosity, are taking us to new frontiers. No one fully knows or can explain why lift happens2, but we're making progress by taking into account downward deflection, pressure differences, and a detailed look at the flow.
Basic Attributes of Lift
That was a lot on lift, but I'm still curious. Lift results from pressure differences and depends on a couple of things, such as angle of attack, airfoil shape, air density, and airspeed.
Angle of attack
The angle of attack is the angle at which the chord of an airfoil (airplane wing) meets the relative wind.
For instance, a symmetrical airfoil will generate zero lift at a zero angle of attack. But as the angle of attack increases, the air changes direction through a larger angle, velocity increases, and more lift is created.
Airfoil Shape
With what we just learned, which airfoil do you think is better?
The lift force generated by an airfoil depends on the shape, especially the amount of camber (curvature). If you increase the camber, you generally increase the maximum lift. This is interesting because it also explains why planes can fly upside down. It's not so much about the upper surface is curved and the lower surface being flat; it's about the air moving through an angle. Air moving through an angle is what matters.
Flow Conditions and Air Speed
Fluid density, speed of flow, and viscosity affect lift, and temperature affects density. Lift is proportional to the density of the air and approximately proportional to the square of the flow speed.
Perfect Airfoil?
There is no "perfect" or "most optimal" shape for a wing airfoil, and it all depends on what you're trying to achieve, so every design is based on the function of the aircraft.
Different designs, different purposes. The Dragonfly wing is the most impressive. Nature is the ultimate designer.
The design process to determine the appropriate shape is, of course, math-based. Engineers use the lift coefficient to measure the amount of lift obtained from a particular airfoil shape. If the lift goes up, so does the dynamic pressure and the wing area. The lift equation:
Drag
If we talk about lift, we have to talk about drag. Drag is easy to understand because you experience it every time you drive and roll down your window. When you take your hand out the window, that force pushing your hand is … drag.
Drag can also be called air resistance. Like lift, drag is also a force that acts opposite to the object's motion. Drag is generated by the difference in velocity between the object and the fluid. In this case, air and airplane or air and your hand.
We have two types of drag: 1) parasite drag and 2) induced drag.
The first drag is called parasite because it doesn't aid flight. Parasite drag is the combination of form and skin friction drag.
Form drag is what we're most familiar with because it is the resistance to the motion of an object through the air. This drag depends on the shape of the aircraft, and form drag is due to the size and shape of the body.
Skin friction drag is the friction between the air molecules and the aircraft's surface. This friction depends on the properties of both the solid and the gas. For instance, a smooth surface produces less skin friction for the solid than a roughened surface. In contrast, the gas depends on the air's viscosity and the Reynolds number, which is the ratio of inertial forces to viscous forces.
The generation of lift causes induced drag, and it is also called "drag due to lift" because it only occurs on lifting wings. This drag occurs when the lift distribution is not uniform on the wing but varies from root to tip.
There are more types of drag, such as wave drag which appears for supersonic aircraft.
Similar to lift, we also have a drag coefficient proportional to dynamic pressure and the area on which it acts. While engineers try to have a lift coefficient as high as possible, engineers design the drag coefficient as low as possible, and low drag coefficients make the aircraft more efficient.
Next, we have the last two forces of flight: weight and thurst.
Weight
The heavier a plane is, the more lift it requires, and it may also require thrust to accelerate. Weight distribution is also essential. The weight must be balanced for small aircraft, as too much weight on either end of the plane can make it unstable. Weight can be calculated with Newton's second law:
Flying involves two significant problems: 1) overcoming the weight and 2) controlling the object in flight. Both are heavily related to the object's weight and the location of the center of gravity.
Thrust
Thrust is the force that opposes drag, and thrust needs to be greater than drag to the plan to accelerate and take off. Thrust is all about propulsion, which involves all kinds of science, from thermodynamics and fluid mathematics to aerodynamics and physics. This is a force that Newton's second law can also describe:
In simple terms, thrust is produced by accelerating a mass of air. But the propulsion system used on an aircraft may vary in different airplanes, and each plane has thrust slightly differently. The four principal propulsion systems are the propeller, the turbine or jet, the ramjet, and the rocket.
Thrust Equation
Although thrust can be described by F = ma, that’s not enough,
Therefore, we need to learn about the thrust equation, which describes how the amount of thrust depends on the mass of the air and how much of it is accelerated through the engine.
But first, how is thrust generated? Newton's Third Law can explain this as for every action, there is an equal and opposite reaction. Here, the air is accelerated to the end, and the engine and the airplane are accelerated in the opposite direction. To expedite the air or the gas, you need a propulsion system, which we'll learn about soon.
This one is a bit mathy but worth learning as it's one of the essential things from flying simple airplanes to launching big rockets.
Important Ratios
If you start learning about flying forces or planes, you will start to people throw around a bunch of ratios so let’s talk about them briefly.
Lift-to-Drag Ratio
It's the amount of lift generated by a wing compared to its drag. It's determined by dividing the lift coefficient by the drag coefficient, and this ratio indicates airfoil efficiency.
The lift-to-drag ratio is called the L/D ratio, pronounced "L over D ratio." An airplane has a high L/D ratio if it produces a significant lift or a slight drag, and if you're cruising, trust is equal to drag. Aircraft with a high L/D ratio can carry a large payload for long periods over a long distance.
LD=CLCD
Both lift and drag coefficients are determined experimentally using a wind tunnel. A "good" lift-to-drag ratio is 30:1.
Thrust-to-Weight Ratio
It's directly proportional to the acceleration of the aircraft. An aircraft with a high trust-to-weight ratio has high acceleration, indicating propulsion efficiency.
The thrust-to-weight ratio can be described like this:
FW=ThrustWeight=mamg=ag
Where:
a = acceleration
g = gravitational acceleration
m = aircraft mass
If the thrust-to-weight ratio (TWR) or (FW) is greater than 1.0, the aircraft can accelerate vertically like a rocket.
Fly, fly, and fly!
Prior to writing this essay, I didn’t understand how complex forces like lift could be and how rewarding understanding what ratios such as thrust-to-weight ratios mean. This is the beauty of teaching yourself anything you want because you can go explore and learn anything you want to discover.
“I don’t know X, I will learn X” is the entire process.
Curiosity is the limit and curiosity is limitless.
If you’re still curious, I’d recommend you read:
Yesterday I rewatched Top Gun for the second time, and I have to admit, if I hadn’t watched Top Gun, I would not have been as interested in flying things as I have been.
In fact, this image was the start of what became a 100+ document with almost 20,000 words and most importantly a fascination into all things flying!
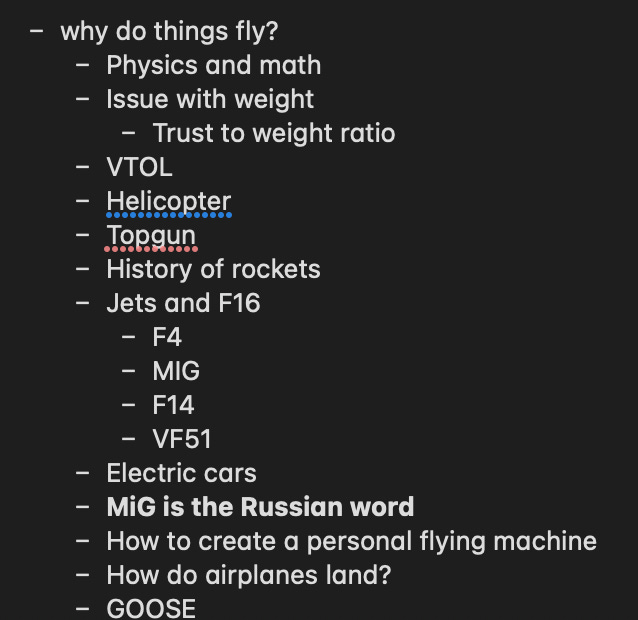
See you next Sunday,
Juan David Campolargo
From https://arxiv.org/pdf/1410.6753.pdf